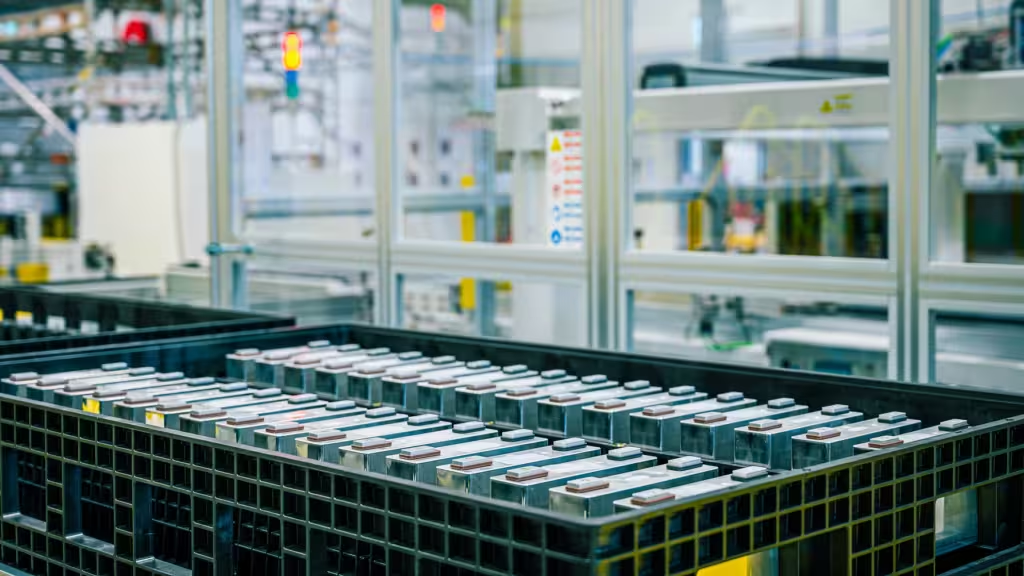
Callum McGuinn, partner at European intellectual property (IP) firm Mewburn Ellis, rounds up the major advancements in battery cell technology that BESS industry sources should be aware of.
Advancements in battery technologies are highly significant for the large-scale energy storage systems (ESS) industry. Key developments to monitor include cell longevity and degradation management, energy density, fire safety, and non-lithium chemistries.
Enjoy 12 months of exclusive analysis
- Regular insight and analysis of the industry’s biggest developments
- In-depth interviews with the industry’s leading figures
- Annual digital subscription to the PV Tech Power journal
- Discounts on Solar Media’s portfolio of events, in-person and virtual
Cell longevity and managing degradation
Degradation reduces a battery’s ability to store charge and deliver power. Improving cell longevity will mean that batteries will need to be replaced less frequently, leading to lower costs and a smaller environmental impact.
Charging practices significantly affect the lifespan of electronic devices. A state of charge between 20% and 80% is optimal, and overcharge or deep discharge can reduce longevity. Exposure to extreme temperatures can also accelerate degradation.
The same of course applies to ESS, which use battery management systems (BMS) to manage charging and discharging cycles to maintain optimal operating conditions, and thermal management systems maintaining optimal operation temperature. These thermal management systems often use phase change materials and heat dissipation technologies to manage the flow of heat.
Recent innovations involve the use of AI-driven BMS to improve accuracy and efficiency. Traditional mathematical models have had difficulty understanding the complex, non-linear behaviour of batteries, and as such are limited in their accuracy. Data demonstrating the numerous external influences on batteries allows AI to recognise patterns and correlations, understanding these better than we did previously.
Through this deeper understanding the BMS can accordingly adjust the battery operation to minimise degradation.
According to power solutions provider MPS: “The algorithms could change their parameters to maximise battery lifespan if they notice a trend of daily deep discharges followed by nightly slow charging. Similar to this, they may suggest preventative maintenance procedures or control measures to lessen the negative impacts of heat on battery life if they notice a pattern of operation in hot environments”.
Energy density
Energy density per se is not a controlling factor for stationary battery storage. Instead, what matters is the areal energy density achievable on the plot of land where the installation is based. Although the energy density of a battery feeds into the overall areal density, it is not the only factor.
Assuming batteries could be stacked vertically, then the areal energy density could in theory be easily multiplied. However stacking of lithium-ion battery systems could present safety issues, not least due to flammability (see below). Alternatives such as redox flow batteries (see below) would more easily facilitate upward scaling of installations. Moreover, large-scale lithium-ion battery installations tend to be located in rural areas where outward growth of the installation is not so problematic.
Assuming we do stick with lithium-ion, a change in anode material from the traditional graphite anode to silicon could deliver greater energy density. While graphite has a durable structure which means it is resistant to swelling and physical damage, there is a limit to the overall energy density that can be achieved with lithium-ion batteries using this material.
Silicon can hold 10 times more lithium ions on a per-mass basis than graphite. However, this technology is still in its early stages, and while it offers increased energy density, several challenges must first be addressed. One significant issue is the expansion of silicon to three times its original volume when it absorbs lithium ions.
Solid-state batteries have the potential to offer greater energy density than the traditional lithium-ion battery. However, further innovations are needed before solid-state batteries become the norm, as their production methods are currently complex and expensive.
Fire safety
When batteries fail, they can release a significant amount of energy very quickly, which can result in thermal runaway, eventually risking a battery fire or explosion. If the energy density of batteries increases, this could allow even more energy to be released rapidly, escalating the risk of a severe thermal runaway. Consequently, fire safety will become an even more important consideration as batteries become more powerful.
Safety mechanisms such as BMS prevent overcharging, overheating, and over-discharging of batteries, which reduces the risk of scenarios that could lead to thermal runaway. Additionally, thermal management systems ensure that batteries remain within safe operating temperatures, further enhancing safety.
Innovative companies are developing ways to arrest thermal runaway, such as the Thermal Runaway Fluid developed by LifeSafe Technologies. The fluid draws on both an endothermic reaction and the suppressing of oxygen to bring thermal runaway under control.
Solid-state batteries reduce fire risk by replacing flammable liquid electrolytes with a stable, non-flammable solid, which significantly reduces the risk of a fire. Redox flow batteries are another promising alternative to lithium-ion with a much-reduced risk of fire.
Non-lithium chemistries
As demand for lithium increases, there is a growing interest in alternative battery chemistries to alleviate the pressure on supply.
Redox flow batteries, especially vanadium redox flow batteries (VRB), offer the most promising alternative to Li-ion, and are already in use in stationary storage systems – in 2023, the UK based company Invinity was awarded £11 million to build a 7MW/30MWh VRB for the UK grid.
Since VRBs don’t lose capacity over time, they don’t need to be oversized at the time of installation. The water-based electrolytes are non-flammable, offering a safer alternative to Li-ion. VRBs have more than double the lifespan of Li-ion batteries, offer a wider range of operational temperatures and provide independent control over power and energy (the energy stored by a VRB is dictated by the size of the electrolyte tanks, whereas the power delivered is determined by electrode area).
Like lithium, vanadium is a scarce resource, which has led to recent research into alternatives. Organic compounds such as viologens are affordable and could replace vanadium. Current research is focused on improving viologen solubility to boost energy density.
Another alternative is the sodium-ion battery. Sodium is a more abundant, lower cost, less flammable, and more sustainable material than lithium. However, sodium batteries have a lower energy density, as well as a reduced cycle life compared with Li-ion.
As discussed above these are both important factors for ESS, and further innovations are needed to enable sodium batteries to more fully replace lithium. Nevertheless China has launched a 50MW/100MWh sodium-ion ESS, with a plan to eventually double its power and capacity, which demonstrates how sodium is a viable alternative.
Zinc batteries are another alternative, utilising a non-toxic, abundant element which has a lower environmental impact than lithium. On the flipside, zinc has a lower energy density and reduced life span compared to lithium. Zinc-air, zinc-ion, zinc-manganese and zinc-carbon batteries are all possible, with current research directed to improving energy density and cycle life.