Paul Verrill, director of energy data analysis & consultancy firm EnAppSys, explains how renewable energy generation, with the integration of smart grid technologies and efficiency energy storage systems, can create a sustainable power system for the future. This article first appeared in E-S.N’s ‘Storage & Smart Power’ section of Solar Media’s quarterly journal, PV Tech Power 22.
Electricity markets across Europe are seeing the growth of renewables within their mix, with levels of generation from renewables overtaking those from fossil fuels across Europe in 2019. Whilst the pace has slowed in recent years, each new year sees records being broken in respect of the proportion of electricity demand met by renewable energy.
Enjoy 12 months of exclusive analysis
- Regular insight and analysis of the industry’s biggest developments
- In-depth interviews with the industry’s leading figures
- Annual digital subscription to the PV Tech Power journal
- Discounts on Solar Media’s portfolio of events, in-person and virtual
Predominantly the growth of renewable generation is coming from increased levels of generation from wind farms – and historically from solar sources – although hydro remains the primary source of renewable generation in European markets.
This growth can lead to problems in security of supply, but the most significant are maintaining stable operation of power grids that were designed for large centralised
thermal power stations. This also means ensuring that there is enough generation to meet demand in periods when output from renewables is low.
In most countries in Europe, the solution to date has been to introduce reserve and/or availability payments via capacity payment mechanisms to supplement the income of existing power stations and incentivise the build of new power stations that are able to meet demand in periods when renewable output is low. These mechanisms in the main provide support to thermal power stations and enable the management of the energy transition but slow down the closure of carbon-emitting assets.
Drivers created by these mechanisms (which are often closed to high-polluting stations) and the European carbon market are increasing the switch from coal to gas but do not yet drive transition to a ‘net zero’ world.
Some markets, Ireland and Great Britain, for example, are approaching the point where renewables are exceeding 50% of the fuel mix in some periods, which causes challenges for grid operation.
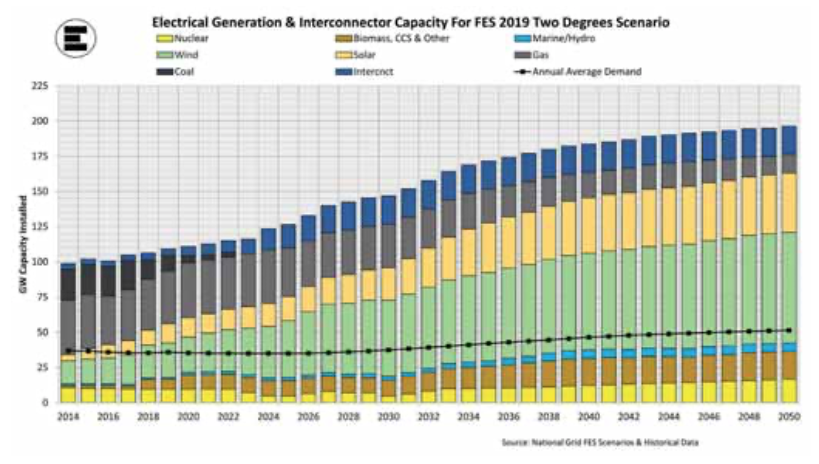
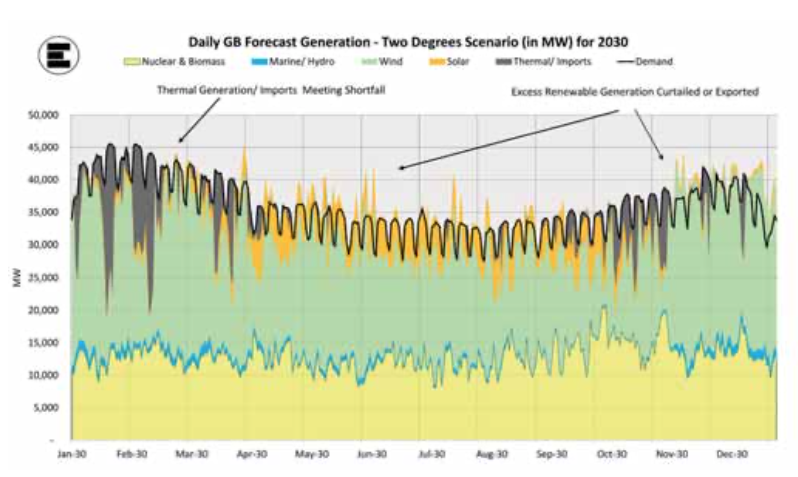
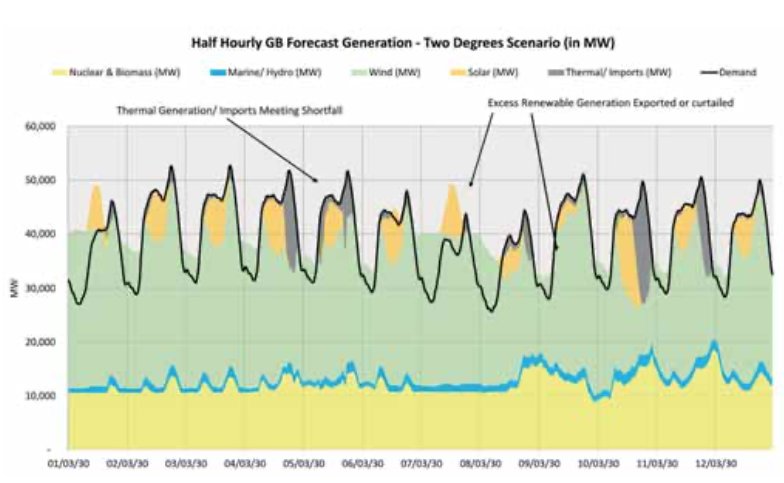
Some of the transmission system operators (TSOs) publish forward energy scenarios to look at potential fuel mixes going forward, most notably Great Britain’s National Grid, which publishes its Future Energy Scenarios. The chart in Figure 1 shows its high renewable scenario with the forecast of average demand plotted on it. This graphic highlights that installed renewables will have the capability at peak output to more than exceed required demand and, whilst export via interconnectors can deal with this excess, it is likely that there may be excess in neighbouring countries as well.
The charts in Figures 2 & 3 show a projection of GB generation fuel mix in 2030 and for a summer two-week period. These have been produced using the EnAppSys forward model and assumes National Grid’s 2 Degree Future Energy Scenario. Periods of excess renewable generation and periods of low renewable generation can be seen. In these scenarios, thermal generation is used to meet demand in the shortfall periods and excess renewable generation is curtailed (i.e. turned down).
Energy storage systems and smart grid technologies are ideally placed to work within this type of fuel mix to reduce the use of fossil fuels, limit the curtailment of zero-carbon generation and contribute to grid stability services – overall, providing progress to meet the net zero target.
The smart grid technology that provides these benefits has features that enable it to measure in real-time energy usage, be combined with some model determining future demand at the point of metering and have some level of control either through demand user action or automatically to change that energy use. Certain demand sites that have generation and/or storage behind the meter would also be able to provide export as part of the response.
To truly decarbonise would require differing types of energy storage that offer a combination of short- term, medium-term and longer-term zero-carbon energy storage technologies. Periods when renewable output is low, or high for extended periods, would not be able to be managed by smart grid technology and/ or short duration energy storage.
In addition, when renewable output is low for sustained periods (i.e. days) then long-term (strategic reserve) storage would be required.
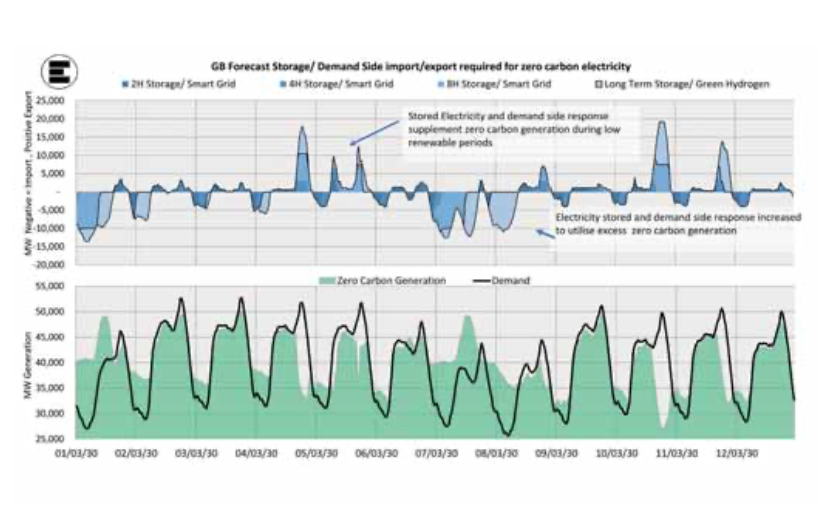
Figure 4 shows the previous fuel mix with a combination of energy storage and smart grid technology varying both demand and supply to maximise the use of zero-carbon generation.
A combination of front-of-meter storage and behind-the-meter storage enables the demand curve to be matched more closely with the generation curve. Peaks of renewable generation are either stored for use in periods of high demand or else peaks of demand are flattened by moving non-time-critical electricity usage to periods of high renewable output. This is already happening but not yet at the scale that is anticipated.
These charts use GB market forecast for 2030 with a high renewable fuel mix and assumes no interconnector export of excess renewable energy.
To enable all zero-carbon energy that could be produced by a combination of storage and demand response, that is around five times the import capacity of current GB storage and represents more than 1,000 times the storage capacity. Whilst the scenario used is the highest renewable build scenario, it illustrates the requirement for a significant build of low-cost ‘bulk’ storage capacity in a market with high wind and solar generation mix.
Technologies
Currently the majority of energy storage deployment is via lithium-ion batteries, with the advances driven by growth of portable electronic devices feeding into the automotive sector and now driving improved batteries into grid-scale energy storage.
The GB market has perhaps seen the greatest deployment of batteries in the last five years through the construction of a number of lithium-ion battery projects with close to 1GW in operation or being completed. It should be noted that in the main these projects have been constructed to meet the former issue with increased renewable deployment, i.e. grid stability.
The installed projects to date are typically fast (sub-one second), short duration (less than one hour) projects and are designed to provide frequency support to maintain supplies. The primary reason for this is that the current capital cost of this technology and degradation means that it requires a higher price differential between import and export than is currently the case in European electricity markets for them to be economic in pure time shifting/pure arbitrage operation. A premium for their speed is currently required but with falling capital costs and improved technical performance this is likely to change.
Lithium-ion batteries are suited to deployment at domestic scale, and commercial products already exist, such as the Tesla Powerwall. With the growth of EV vehicles, a battery installation can share key infrastructure (inverters etc.) with charging infrastructure, making them complementary and delivering powerful smart grid capability.
Flow batteries, typically non-solid- state energy storage devices, are being developed but the level of R&D spend is significantly lower than for lithium ion as they do not yet have the same consumer application as lightweight, energy-dense storage devices. The technology has the capability for long-duration storage and for certain technologies employing rotating machinery to provide grid inertia, reactive power and fault tolerance stability services to grid, which the majority of grid systems in Europe were designed for.
Going forward, the potential for hybrid storage involving solid state batteries for speed, coupled with flow batteries for duration and potentially with certain technologies to provide grid inertia is an option for the future. Technology-wise, all of the elements are there, but the drivers are not as developed for this combination of abilities.
The oldest storage on the system both in GB and across Europe is pumped hydro. These are typically fast-acting (sub-two-minute assets), long-duration assets, offering anywhere from four hours of sustained output to more than 12 hours for some projects in planning. Across Europe the average age of stations is around 30 years and whilst new units are coming online it is rare as they require large upfront capital investment and therefore ideally long-term revenue certainty.
A means of energy storage that would also drive decarbonisation of heating systems as well as using existing generation infrastructure is hydrogen, ideally produced from excess renewable energy or from carbon capture technologies. This has the advantage of being able to use established gas storage infrastructure and gas-fired generation assets to provide flexible and, more importantly, sustained generation in periods of low renewable output, which simplifies the journey towards net zero. In the case studies above, the production, use and storage of green hydrogen in existing natural gas storage and transmission infrastructure would enable the decarbonisation of thermal generation and heating.
Smart grid capability on the demand side is growing across Europe with the roll-out of smart meters. ‘Agile’ tariffs now exist in GB where price signals are communicated to consumers; they pay on a real-time price basis and this drives behaviour on their use of energy.
Barriers
The primary barrier to the deployment of energy storage and smart grid technology to deliver a sustainable power system is the level of investment required. The development needs certainty of revenues for an investment community and needs to be affordable for the consumer in the case of domestic applications.
Mechanisms for rewarding energy storage and smart grid technology are not well developed. Most of the investment in this technology requires the major proportion of its revenue to be obtained through merchant markets, which can be difficult to finance against. In the consumer market, the smart meter roll-out is assisting the market but the use of this capability is still limited.
As investment in storage technologies increases and the more it acts to enable the transition to net zero, the lower the returns for the assets if operating on pure merchant returns. The reason for this is that they derive their revenue from the price spread between periods of high demand and low demand; and the more demand is matched to generation, the lower this price spread. For assets also deriving revenue from providing grid stability services, the amount of installed assets required to balance supply and demand could create excess competition for the provision of these services, driving prices very low.
Enablers
Recognising certain medium/long-term storage assets as grid infrastructure would enable TSOs to build and/or procure storage as part of their overall network investment programme or else to have support through cap and floor mechanisms in a similar way to interconnectors. This would help the more strategic deployment of energy storage to either reduce transmission flows or cover long duration reserve.
In some markets, further deployment of weather-dependent renewable technologies requires the developers to build a proportion of energy storage to match and support their technologies. This creates a market for these projects and a share of the support given to renewables.
Currently, processes to support renewable/zero carbon can be technology-targeted and centrally the government retains the requirement to balance out any of the negative aspects of the technology deployed. For example, in GB offshore projects
are supported by the CfD (Contracts for Difference) mechanism, the government then has a capacity market mechanism to counter the impact of high renewables and the TSO procures alternative flexible generation to meet grid stability requirements.
There is the potential to extend this, and tender processes that seek to procure zero or low-carbon electricity generation able to match supply and demand would allow developers to team up and innovate to deliver solutions with a portfolio approach. This would find the most complete solution to delivering net zero power and could even include an element of demand response within it. Smart grid-enabled consumers could help a group of technologies to deliver zero carbon dispatchable generation similar to a nuclear project.
Capacity market mechanisms that target energy storage could alternatively provide the type of investment support that is driving investment in conventional generation. Currently, energy storage is positively discouraged in capacity mechanisms through the way in which derating factors are calculated.
Conclusion
Energy storage and smart grid technologies are developing rapidly and projects that provide positive benefits to the system are being deployed across Europe. The potential contribution they can make to a sustainable energy supply is recognised, and most system operators and governments are encouraging it through roll-out of smart meters and modification of system operation to recognise the changing fuel mix.
Despite this, the progress made so far is arguably ‘behind the curve’, as evidenced by the issues identified in the GB power cut of August 2019.
In our view, the market structures to allow energy storage and smart grid technologies to fulfil their potential and play their part in delivering a sustainable energy supply in Europe are not there yet. Whilst there are initiatives, trials and schemes that are supporting investment, it is not clear that these are scalable and that a consensus exists for the European market to adopt a whole-system approach. The emphasis currently is on the transition from the old world to the new and not so much on what the end system ideally would look like. It may well be that we must decide on a destination before a truly effective map can be made of how to reach that point.
This article first appeared in E-S.N’s ‘Storage & Smart Power’ section of Solar Media’s quarterly journal, PV Tech Power 22, available now as a free download from the PV Tech Store.
Cover Image: Cleator, a 10MW battery park in the UK. Credit: VLC Low Carbon / NEC.