A future where 100% of the world’s electricity comes from clean energy sources isn’t just wishful thinking – it’s mandated by the hard realities of climate change. Getting energy from fossil fuels is a hard habit for humanity to break, but the good news is that momentum finally is on the side of renewable energy and energy storage. Renewables targets at every level of government, new capacity market rules being implemented by grid operators, and the availability and cost-effectiveness of long-duration energy storage resources are all helping create a major paradigm shift in the energy industry.
Across the United States, several states and more than 100 cities have adopted ambitious 100% clean energy goals. Xcel Energy made a landmark announcement in late 2018 as the first US utility to commit to fully providing clean energy with a pledge to supply 100% carbon-free electricity by 2050 across its service area in eight US states.
Enjoy 12 months of exclusive analysis
- Regular insight and analysis of the industry’s biggest developments
- In-depth interviews with the industry’s leading figures
- Annual digital subscription to the PV Tech Power journal
- Discounts on Solar Media’s portfolio of events, in-person and virtual
Europe has made even greater progress. Most European countries have set similar renewables targets, and some have made significant headway. Germany gets 36% of its electricity from renewable sources, Denmark gets over 50% and Iceland generates the most clean electric-ity per person on earth, with almost 100% of its energy coming from renewables.
The quantum leaps we’re seeing in the market are also possible because the cost of renewable energy is on par with fossil-fuel generation. The levelised cost of electricity (LCOE) for utility-scale solar fell 85% from US$350/MWh in 2009 to US$50/MWh in 2017, and according to the National Renewable Energy Laboratory, is expected to drop to US$37/MWh by 2050. The wind industry saw similar price drops, with the mean wind energy LCOE dropping to US$45/MWh in 2017.
Competitive prices are helping to spur unprecedented levels of renewables deployments. The U.S. Energy Informa-tion Administration projects that from 2020 to 2050 utility-scale wind capacity will grow by 20GW and utility-scale solar photovoltaic capacity will grow by 127GW in the United States alone. With these market drivers making renewables smart business, it’s clear that the march toward 100% clean energy is much more than a political movement.
To successfully incorporate ever greater amounts of renewable energy into the grid while maintaining reliability, energy storage assets will need to be capable of steadily injecting electricity into the grid for much longer periods of time than is currently the case. Grid operators are turning to long-duration energy storage (more than four hours) to help improve power generation economics, balance the grid and increase reliability. The promise is to enable renewables to become baseload power – a feat that is nearer in the timeline than many believe.
Cornerstone to 100% renewables – long-duration energy storage
One challenge in adding more renewable energy sources to the power grid is figur-ing out how to deal with the intermittent power generation from these sources. Consumers, of course, demand reliable power. But if the whole system is lacking enough generation, short-duration energy storage resources simply would not be able to sustain sufficient power output for a long enough period of time to meet power demands. The good news is that with at least eight hours of capacity, energy storage resources are equivalent in performance to – and could replace – a fossil fuel power station.
Such long-duration energy storage resources can also support the electricity transmission and distribution systems while providing additional security of supply. The benefit to the grid – and more importantly, to our planet – is that longer-duration energy storage will enable grid operators to maximise renewable energy penetration without needing fossil fuel generation to make up for intermittency.
This makes replacing gas peaker power plants with a combination of solar, wind and energy storage a viable reality and truly sets the stage for a future where 100% of the world’s electricity comes from clean energy sources.
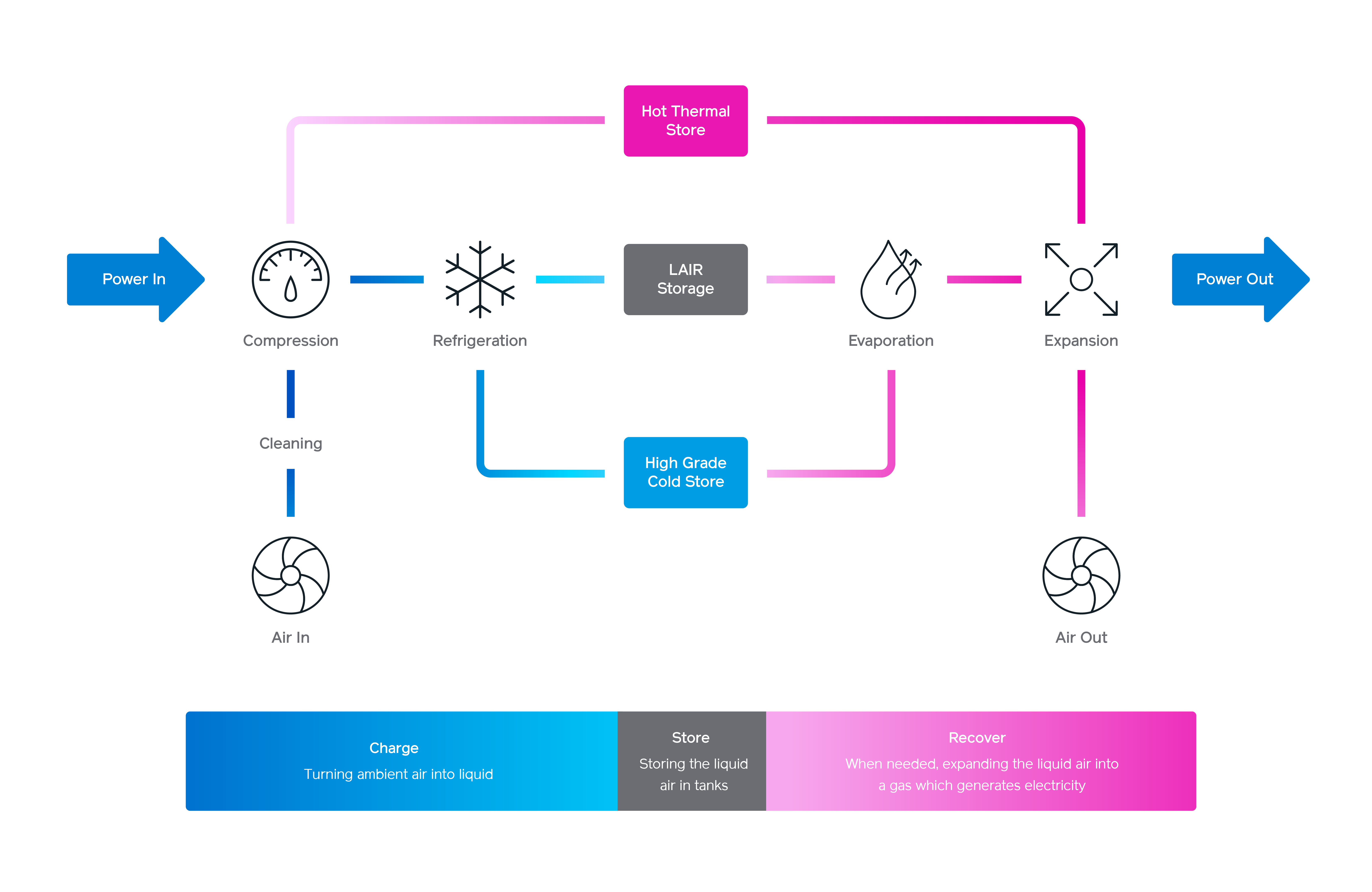
What options are available today?
Many energy storage technologies are already in use, but each presents its own challenges for grid-scale, long-discharge storage. Pumped hydro and cavern-based compressed air storage have been used effectively for very large-scale energy storage projects, but both have siting constraints. Pumped-hydro requires close proximity to two large bodies of water with hundreds of feet of drop between them; compressed air storage calls for injecting compressed air into airtight, geologically stable structures, such as caverns or depleted oil or gas fields, which can be difficult to find. Lithium-ion batteries have been used for stationary energy storage applications, due to their high energy density and relatively low upfront cost. However, the longer the discharge period for a given capacity, the more batteries you need. At the hundreds of megawatts scale, the economics for two or more hours of discharge is a challenge.
Lithium-ion batteries are also tough on the environment; after a relatively short life, their components are difficult to recycle. They also can pose a fire risk if not properly managed, and they rely on diminishing metal resources (such as cobalt) which come with mining risks. Flow batteries are often more economic than lithium-ion, and they don’t degrade as quickly. However, there is a cost to scaling to higher power capacities. Like all batteries, they are limited in their ability to reliably provide the synchronous inertia and voltage control in the way that is possible for traditional power plants with spinning generators.
This means that, as more and more solar and wind power is added to the grid, battery storage is limited in its ability to respond to large imbalances between electricity supply and demand while keeping frequency and voltage stable. One emerging, long-duration energy storage option, with the potential to mitigate many of the constraints posed by other systems, is cryogenic energy storage technology.
A versatile, environmentally friendly option emerges
Cryogenic energy storage systems, which use liquid air, are better suited to provide grid-scale storage than pumped hydro-power or compressed air because they are freely locatable systems that can be sited just about anywhere. Cryogenic energy storage plants have a small footprint, don’t use any hazardous materials, have no associated fire risk, and can easily meet strict urban building codes. The technology uses liquid air as the storage medium by cooling ambient air (using conventional industrial refrigeration) to lower than -270°F (-170 ̊C), which results in a 700-fold contraction in its volume from gas to liquid. The process is similar to that used to liquefy natural gas, but because these cryogenic systems use only air, the entire charge/discharge cycle is completely free of carbon emissions.
The liquid air is stored in conventional insulated tanks at low pressure (such as those used for liquid oxygen or nitrogen storage at facilities such as hospitals). When energy is needed, the liquid is warmed and pumped to pressure, then expanded back to gas with a 700-fold increase in volume. The expansion takes place through a standard expansion turbine connected to a generator to generate electricity, thus releasing the stored energy. There is no combustion – the only thing released into the atmosphere is clean air (Figure 1).These cryogenic systems are the only long-duration energy storage solution available today that offer multiple gigawatt hours of storage. That represents weeks’ worth of storage, not just hours or days.
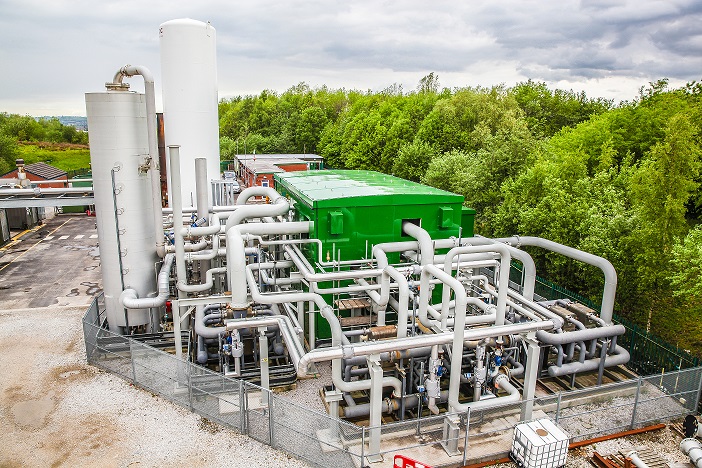
Modernising the grid to remove barriers to renewable power
A critical benefit of this giga-scale storage is that these systems can provide services at all levels of the electricity system—supporting power generation, providing stabilisation services to transmission grids and distribution networks, and acting as a source of backup power to end users. Cryogenic energy storage can help power systems deal with operational limitations that prevent large amounts of variable renewable generators from being integrated into the energy mix at any given time. These limitations are related to the speed at which resources can respond to imbalances between electricity demand and supply. One of the main tasks of grid operators is to keep electricity generation and demand balanced at all times since sustained imbalances result in blackouts and system failures.
Currently, most electricity systems rely on a form of energy storage known as synchronous inertia to help overcome imbalances between electricity demand and generation. Basical-ly, synchronous inertia functions much like shock absorbers on your car that limit the impact of bumps or holes in the road. Synchronous inertia levels will decline significantly in the future as more renewables are integrated, potentially limiting the maximum share of renewa-bles in the overall energy mix. Most forms of non-synchronous generation, including solar PV and wind turbines, rely on power electronics to convert primary energy into electricity.
This kind of generation, while great for the planet, is not as effective at acting as a shock absorber because although the response is very fast, there is not a mechanism that ensures power stability. System operators have defined param-eters that help identify operational limits to the amount of non-synchronous genera-tion that can be integrated into the grid at any given time. In Ireland, for example, grid operators monitor their non-synchronous penetration ratio as a way to estimate the maximum share of non-synchronous generation in the energy mix that can be integrated at any given time without jeopardising system stability. This ratio is currently estimated to be 50%.
To overcome operational limitations and guarantee security of supply, grid operators are developing new network codes and are redesigning their ancillary services markets with the aim to procure synchronous inertial response (SIR) and other auxiliary products. Cryogenic energy storage can provide synchronous inertial response. These systems use motor-driven compressors to liquefy air and charge the energy store, and a turbine-driven synchronous genera-tor to inject power to the grid. This creates a scenario where both the charging and the discharging units of a cryogenic system can provide synchronous inertia. Interest-ingly, both units can run concurrently which can be very valuable.
For example, system resiliency tends to be very weak when electricity demand is at its minimum level and the penetration of non-synchro-nous generation is high. These events occur across the year, normally at night or on sunny summer weekends when people are on vacation. In this scenario, few conventional generators serve system load, reducing system inertia to minimum levels. Under these circumstances fluctuations from renewable generation may create imbalances that need to be resolved more quickly than normal to avoid major system failures, impacting the cost to operate the system.
Managing the costs associated with operating the system under low synchro-nous inertia conditions will entail minimis-ing renewable curtailment and procuring cost-effective system services. Operating the charging unit of a cryogenic energy storage system not only helps avoid curtailment but also contributes to provid-ing synchronous inertia. Additionally, the discharging unit could be operated at its minimum export level or even operated as a synchronous condenser contributing to system inertia and voltage control. This would result in a reduction in the amount of fast reserves needed for frequency and voltage control. Given the operational capabilities of most electricity grids around the world, there is a limit to how much renewable energy can be integrated at any given time while maintaining grid resiliency. Operating the grid with low levels of synchronous inertia would prove to be expensive, especially when demand for electricity is low. This is where energy storage options, such as cryogenic systems that provide synchronous inertia, can help to reduce costs by reducing renewable curtailment. This could be a key element in maximising the benefits of renewable energy integration.
Conclusion
Only with giga-scale, long-duration energy storage will renewables become reliable enough to become the baseload source of power. As utilities better understand the benefits and technical abilities of long duration energy storage, we will see faster integration of renewables to help us get to a 100% clean energy future.
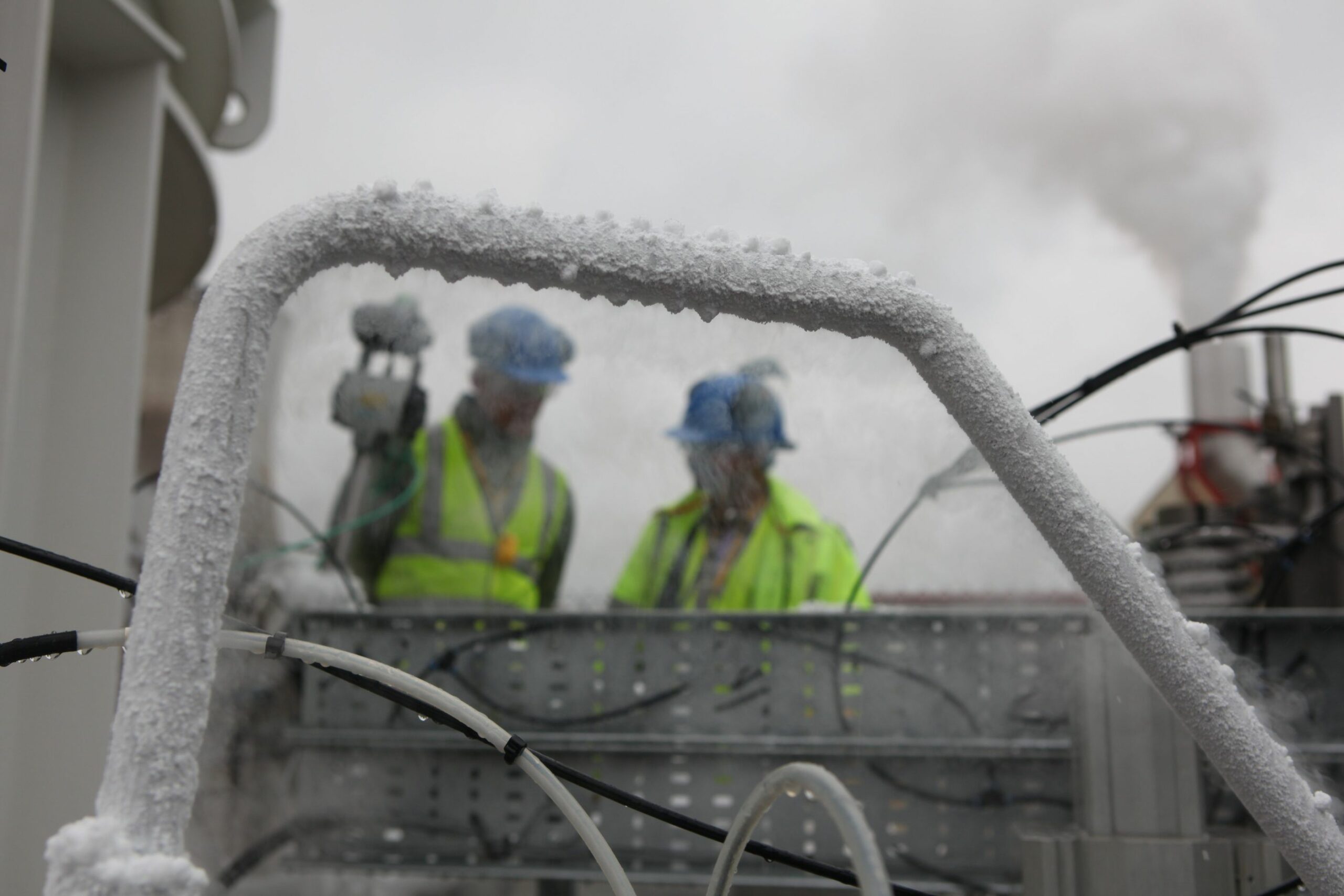
Other applications of cryogenic energy storage
Power generation
• Managing intermittent renewable generation
• Energy arbitrage
• Peak shaving
• Improved heat rate
• Waste heat
Transmission
• Ancillary services
• Transmission constraints
• Inertia services
• Responsible flexibility services
• Voltage support
Distribution
• Reactive power
• Voltage support
• Local security
• Distribution losses
End users
• Power reliability
• Energy management
• Waste heat recovery
• Waste cold usage