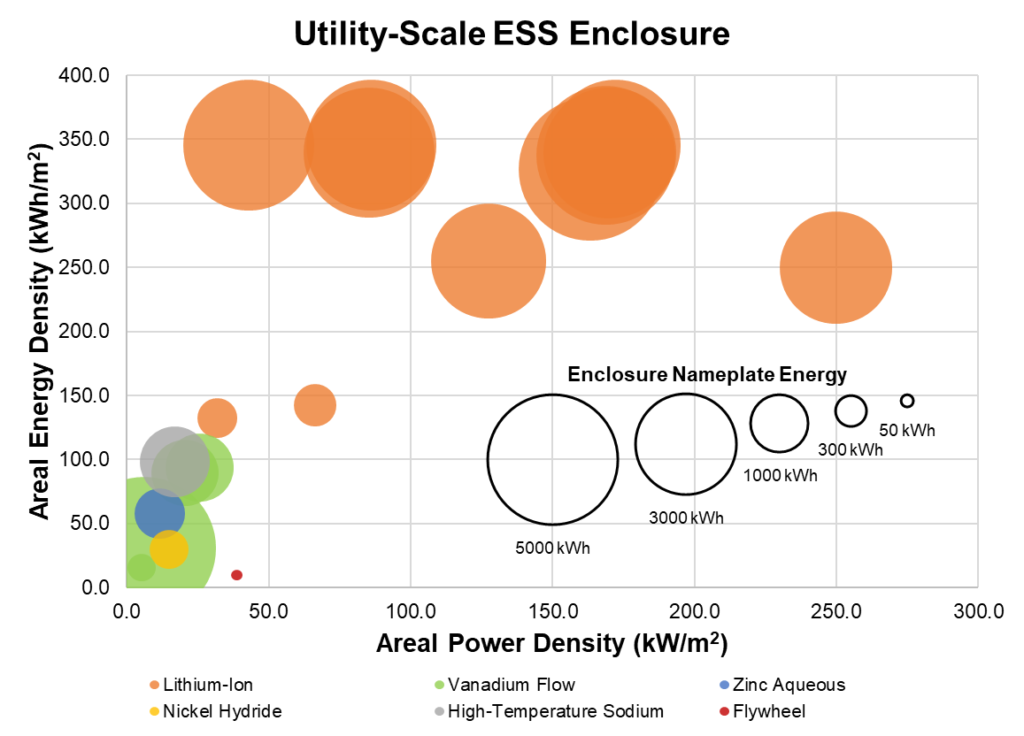
Alternative technologies to lithium-ion are unlikely to oust the incumbent anytime soon, writes Anh Vu of Clean Energy Associates (CEA).
This continues our regular series of exclusive Energy-Storage.news Guest Blog contributions from Clean Energy Associates in 2025, following ‘US battery gigafactories face delays and cancellations amid market uncertainty,’ by CEA energy storage market analyst Anjali Joshi, published in April.
Enjoy 12 months of exclusive analysis
- Regular insight and analysis of the industry’s biggest developments
- In-depth interviews with the industry’s leading figures
- Annual digital subscription to the PV Tech Power journal
- Discounts on Solar Media’s portfolio of events, in-person and virtual
Or continue reading this article for free
Interest in alternative battery chemistries has grown sharply over the past six months. Geopolitical tensions and new tariffs have introduced fresh uncertainty and cost into the battery supply chain, particularly for lithium iron phosphate (LFP) cells imported to the US from China. As a result, many developers are reevaluating their options and exploring technologies that don’t rely on lithium.
Sodium-ion batteries, flow batteries, and a wave of emerging chemistries have drawn attention as potential challengers. But excitement should not be mistaken for readiness. Despite growing interest and years of research, no alternative chemistry is close to displacing lithium-ion for utility-scale energy storage at scale in the near term.
Why lithium still dominates
To understand why lithium-ion remains the standard, it’s important to consider two critical performance factors: energy density and power density.
Energy density measures how much energy a battery can store in a given volume or weight. Power density describes how quickly that energy can be delivered. Lithium-ion batteries, particularly LFP, strike a compelling balance on both fronts.
This balance, combined with lithium-ion’s long commercial track record, has positioned it as the default choice for grid-scale energy storage. Cost reductions driven by electric vehicle (EV) and consumer electronics markets have helped further solidify its position. Manufacturers now have decades of experience fine-tuning lithium-ion systems, from electrolyte additives to thermal management.
Rather than abandoning lithium-ion, most ongoing innovation is focused on pushing it even further through new cell formats, updated materials, higher energy densities, and faster charging capabilities. These improvements are incremental but reliable and build on well-established supply chains and infrastructure.
A long list of contenders—and long odds
Alternative chemistries are not a new idea. Over the past 15 years, numerous technologies have promised improvements on lithium-ion’s combination of strengths: cycle life, cost, manufacturing scale, environmental impact, and power and energy density. These include flow batteries, aqueous systems, flywheels, compressed air, and liquid metal batteries, to name a few.
Few energy storage technologies have reached commercialisation. Examples include pumped hydroelectric, compressed air, and flow batteries. These technologies lag behind lithium-ion due to limitations in energy density, manufacturability, efficiency, maintenance, cycle life, and cost. Improvements to these alternatives often remain confined to lab research, as it is difficult to demonstrate that they could fundamentally and economically outperform lithium-ion.
Sodium-based batteries are one of the more advanced alternatives currently under discussion. Sodium is abundant and inexpensive, and sodium-ion batteries operate on principles that seem similar to lithium-ion at first glance. But sodium’s larger atomic size introduces cascading technical challenges — affecting everything from electrode design to electrolyte formulation and separator compatibility.
Sodium-ion is not alone. Other novel chemistries—such as zinc, aluminium, or magnesium-based systems—are actively being researched, but each brings its own set of trade-offs, ranging from low round-trip efficiency (RTE) to high degradation rates.
The scaling problem
Even if a new chemistry shows promise in laboratory testing, scaling it into a commercial product is a long and uncertain process. Storage developers are open to small-scale demonstrations of new technologies—especially in community storage projects or with utility cooperatives—but that interest doesn’t immediately translate to large procurements.
Utilities, in particular, are cautious. Any new system must demonstrate long-term reliability, safety, and cost-effectiveness. These qualities can only be proven over time, typically through multi-year field deployments and detailed performance data.
Beyond the upfront price of a battery system, utilities and developers look closely at lifecycle cost, including degradation, auxiliary loads, and efficiency losses over time. Lithium-ion systems are well-understood in this respect; newer chemistries are not.
Even if a new battery checks all the performance boxes, it must still overcome manufacturing barriers. Scaling a supply chain, building out production facilities, and achieving quality control at volume can take years.
Why lithium isn’t going away soon
In practice, lithium-ion’s dominance is self-reinforcing. Developers sign 20-year contracts for storage systems, and lithium’s track record makes it the most bankable option. System owners plan for augmentation—the replacement or addition of battery modules midway through a project’s life—and few are willing to gamble on mixing chemistries within a single system.
Even companies that are actively investing in alternative chemistries often continue to deploy lithium-ion in the field. It’s not a lack of confidence in the new technology, it’s a recognition of where the market stands today.
As history shows, lithium-ion itself took decades to mature. From its early use in consumer electronics to its rise in EVs and grid storage, the technology advanced step by step—from bench top experiments to kilowatt-scale pilots to full-scale production. Alternative chemistries are likely to follow a similar timeline.
A path forward but not a shortcut
The future will not be all lithium. For certain applications—such as long-duration energy storage (LDES), harsh climate conditions, or domestic supply chain alignment—new chemistries may eventually offer real advantages. Some are already carving out early niches.
But those gains will come slowly. For the next several years, developers still need to get projects done. That means working within the constraints of what’s technically mature, commercially viable, and reliably available today.
That doesn’t mean standing still. Developers can—and should—continue to evaluate new technologies, monitor pilot deployments, and plan for a more diverse future.
Meanwhile, success in the current market will require flexibility, creativity, and the support of partners who can help navigate the evolving supply risks and procurement challenges for lithium.
About the Author
Anh Vu is a senior energy storage engineer at Clean Energy Associates (CEA), a North American-owned solar PV, green hydrogen, and battery storage clean energy advisory company across the whole value chain, with offices in Denver, USA and Shanghai, China.